The Turkana jet is a low-level, south-easterly wind flowing from Kenya to South Sudan. The jet passes through the Turkana channel between the Ethiopian and East African highlands. The Lake Turkana Wind Power Station (the largest wind farm in Africa, as of 2024) benefits from the semi-permanence of the jet throughout the year.
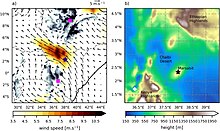
The jet was first presented in scientific literature by Kinuthia and Asnani in 1982 using measurements collected in Marsabit, Kenya.[1] At Marsabit, the wind is strongest around 500–800 m above the ground, and has been known to reach speeds of 50 m/s.[2] There is a variation in jet strength throughout the day, peaking between 00 and 06 UTC.
Causes
editThe factors influencing the Turkana jet and its diurnal cycle are complex. The general wind flow is onto the African continent from the Indian Ocean in association with north-easterly or south-easterly Trade winds, depending on the time of year. However, the strong winds of the jet are a localised feature.
Early modelling studies concluded that the jet forms as a result of orographic channelling (i.e. Bernoulli's principle).[3][4] More recently, it has been proposed that the night-time strengthening of the jet is a consequence of orographic downslope winds (i.e. Katabatic wind).[5]
Characteristics
editScientists have recently returned to take measurements at the Marsabit site[6] where the jet was first measured.[7] The scientists confirmed the existence of a persistent low-level jet. The jet formed every night of the campaign with an average low-level maximum wind speed of 16.8 m s−1 at 0300 local time. The measurements also highlighted a role for the Turkana jet in regional water vapour transport: the mean water vapour transport at Marsabit was found to be 172 kg m s−1.
While direct measurements provide the most reliable estimates of wind in the jet, they are limited in terms of spatial and time coverage. A number of studies have used atmospheric reanalysis data to look at the jet over its full area, and how that may have varied over recent decades.[2][8][5] Findings support understanding of the controls on the jet with higher pressure gradient conditions associated with stronger wind speeds in the jet. In addition, stronger wind speeds are associated with drier conditions in the Turkana channel and surrounding mountains which enhance the night-time downslope winds that feed into the jet.[5] Hartmann additionally highlights the role of temperature gradients in providing a thermal forcing to jet wind[8]
High resolution atmospheric models have been used to simulate the jet,[9] and the jet has even been studied in the coarsest climate models.[10]
Associated weather and climate
editMany scientists have noted connections between the Turkana jet and rainfall. These associations have been studied in the vicinity of the jet.[9][11] In addition, wind-driven effects on Lake Turkana have been investigated.[12]
There have also been investigations of how regional weather and climate relates to the jet. Recently, connections between soil dryness and the jet speed have been presented.[13] That same study highlighted how the soil conditions can enhance the effect of a large-scale atmospheric feature, the Madden-Julian oscillation, on the Turkana jet.
Climate change
editSo far, there has only been one study which looked at the response of the jet to climate change.[9] This studied the pressure gradient force, which could be considered the driving force of the jet. The study found that in two relatively high resolution models (25 km and 4 km grid scales) the force increased with climate change. The result was a stronger wind speed in the lowest parts of the jet, but there was also a slight reduction in wind speeds in higher parts of the jet. The highest resolution model simulated the biggest increase in the pressure gradient.
References
edit- ^ Kinuthia, J. H.; Asnani, G. C. (November 1982). "A Newly Found Jet in North Kenya (Turkana Channel)". Monthly Weather Review. 110 (11): 1722–1728. Bibcode:1982MWRv..110.1722K. doi:10.1175/1520-0493(1982)110<1722:ANFJIN>2.0.CO;2.
- ^ a b Nicholson, Sharon (May 2016). "The Turkana low-level jet: mean climatology and association with regional aridity". International Journal of Climatology. 36 (6): 2598–2614. Bibcode:2016IJCli..36.2598N. doi:10.1002/joc.4515. S2CID 129850035.
- ^ Sun, Liqiang; Semazzi, Fredrick H. M.; Giorgi, Filippo; Ogallo, Laban (27 March 1999). "Application of the NCAR regional climate model to eastern Africa: 1. Simulation of the short rains of 1988". Journal of Geophysical Research: Atmospheres. 104 (D6): 6529–6548. Bibcode:1999JGR...104.6529S. doi:10.1029/1998JD200051.
- ^ Indeje, Matayo; Semazzi, Fredrick H. M.; Xie, Lian; Ogallo, Laban J. (June 2001). "Mechanistic Model Simulations of the East African Climate Using NCAR Regional Climate Model: Influence of Large-Scale Orography on the Turkana Low-Level Jet". Journal of Climate. 14 (12): 2710–2724. Bibcode:2001JCli...14.2710I. doi:10.1175/1520-0442(2001)014<2710:MMSOTE>2.0.CO;2.
- ^ a b c Vizy, Edward K.; Cook, Kerry H. (October 2019). "Observed relationship between the Turkana low-level jet and boreal summer convection". Climate Dynamics. 53 (7–8): 4037–4058. Bibcode:2019ClDy...53.4037V. doi:10.1007/s00382-019-04769-2. S2CID 146494779.
- ^ Munday, Callum; Engelstaedter, Sebastian; Ouma, Gilbert; Ogutu, Geoffrey; Olago, Daniel; Ong'ech, Dennis; Lees, Thomas; Wanguba, Bonface; Nkatha, Rose; Ogolla, Clinton; Gàlgalo, Roba Ali; Dokata, Abdi Jillo; Kirui, Erick; Hope, Robert; Washington, Richard (August 2022). "Observations of the Turkana Jet and the East African Dry Tropics: The RIFTJet Field Campaign". Bulletin of the American Meteorological Society. 103 (8): E1828–E1842. Bibcode:2022BAMS..103E1828M. doi:10.1175/BAMS-D-21-0214.1.
- ^ Kinuthia, Joseph Hiri (November 1992). "Horizontal and Vertical Structure of the Lake Turkana Jet". Journal of Applied Meteorology. 31 (11): 1248–1274. Bibcode:1992JApMe..31.1248K. doi:10.1175/1520-0450(1992)031<1248:HAVSOT>2.0.CO;2.
- ^ a b Hartman, Adam T. (May 2018). "An analysis of the effects of temperatures and circulations on the strength of the low-level jet in the Turkana Channel in East Africa". Theoretical and Applied Climatology. 132 (3–4): 1003–1017. Bibcode:2018ThApC.132.1003H. doi:10.1007/s00704-017-2121-x. S2CID 125433876.
- ^ a b c Misiani, Herbert O.; Finney, Declan L.; Segele, Zewdu T.; Marsham, John H.; Tadege, Abebe; Artan, Guleid; Atheru, Zachary (12 December 2020). "Circulation Patterns Associated with Current and Future Rainfall over Ethiopia and South Sudan from a Convection-Permitting Model". Atmosphere. 11 (12): 1352. Bibcode:2020Atmos..11.1352M. doi:10.3390/atmos11121352.
- ^ Oscar, Lino; Nzau, Mutemi J.; Ellen, Dyer; Franklin, Opijah; Rachel, James; Richard, Washington; Tom, Webb (30 September 2022). "Characteristics of the Turkana low-level jet stream and the associated rainfall in CMIP6 models". Climate Dynamics. 62 (9): 8371–8387. Bibcode:2024ClDy...62.8371O. doi:10.1007/s00382-022-06499-4. hdl:1983/4d625ec4-22a3-43e7-96a3-333cd0da23da.
- ^ Jury, Mark R.; Minda, Thomas T. (April 2023). "Turkana Low-Level Jet Influence on Southwest Ethiopia Climate". Journal of Hydrometeorology. 24 (4): 585–599. Bibcode:2023JHyMe..24..585J. doi:10.1175/JHM-D-22-0134.1. S2CID 257748970.
- ^ Zăinescu, Florin; van der Vegt, Helena; Storms, Joep; Nutz, Alexis; Bozetti, Guilherme; May, Jan-Hendrik; Cohen, Sagy; Bouchette, Frederic; May, Simon Matthias; Schuster, Mathieu (1 April 2023). "The role of wind-wave related processes in redistributing river-derived terrigenous sediments in Lake Turkana: A modelling study" (PDF). Journal of Great Lakes Research. 49 (2): 368–386. Bibcode:2023JGLR...49..368Z. doi:10.1016/j.jglr.2022.12.013.
- ^ Talib, Joshua; Taylor, Christopher M.; Harris, Bethan L.; Wainwright, Caroline M. (July 2023). "Surface-driven amplification of Madden–Julian oscillation circulation anomalies across East Africa and its influence on the Turkana jet". Quarterly Journal of the Royal Meteorological Society. 149 (754): 1890–1912. Bibcode:2023QJRMS.149.1890T. doi:10.1002/qj.4487.